
The situation in Japan is grim. Estimates of the dead or missing — and by now this latter group must be moved into the dead column — is above 25,000 souls. A half-million residents are homeless, with many in danger of starvation since roads and railroads have simply disappeared. Yet the world’s media pays only lip service to the plight of Japanese citizens. It is almost entirely focused on the disabled nuclear reactors and the “leaks” of radiation that have had, and will have, virtually no effect on human health.
In the last few weeks, we have read reports of foreigners scrambling to leave the country, of levels of radioactive iodine in seawater that are seven-and-a-half million times the “legal” limit, and now the news that the recovery of bodies is being hampered because the dead are contaminated by radiation.
Interestingly, many of the expatriates “escaped” to areas where the background radiation was higher, in some cases much higher, than the areas in Japan they were evacuating owing to radioactive releases. An April 1 Bloomberg article by Stuart Biggs and Yuriy Humber gave the current background radiation measurements in Tokyo compared with other areas. Even after the releases in Japan, the amount of background radiation in Tokyo is still below the world average. The article quoted Bob Bury of the UK’s Royal College of Radiologists, “The situation in Japan looks set to follow the pattern of Chernobyl, where fear of radiation did far more damage than the radiation itself.”
Regarding the concentration of Iodine 131 in seawater, one might ask on what basis any legal limit is derived. The “normal” concentration of this isotope in seawater is zero, as only relatively tiny amounts are produced in nuclear reactors, and these for all intents and purposes cease to exist after 90 days because of radioactive decay. This seems to be a case of bandying huge numbers for no other purpose than to create fear — something all too common in journalism these days.
If we analyze for a moment the MSNBC.com story “Japan faces another dilemma: Radiation-contaminated bodies,” we should remember that exposure to radiation does not make one radioactive, e.g., you don’t become radioactive from an X-ray. So any contamination would have had to settle out from the atmosphere onto the bodies. One might ask how the radioactive particles know how to zero in on the corpses and avoid the area that surrounds them.
Even more irony comes from the land of liberals, California — home to Barbara Boxer, Dianne Feinstein, and Governor Jerry Brown — where many residents are fearful of the effects of radioactive particles carried on the winds from Japan. UConn physics professor emeritus Howard Hayden points out in his newsletter, The Energy Advocate, that the joke is on the Californians who are now gobbling down potassium iodine pills to saturate their thyroids in an attempt to block an accumulation of radioactive iodine. The “K” in KI pills is potassium, a small percentage of which is radioactive Potassium 40. In an attempt to avoid barely detectable amounts of Iodine 131, they are ingesting easily measurable amounts of bone-seeking Potassium 40. Actually this radiation won’t bother them either, although the pills are not gentle on the digestive system and give the same symptoms — nausea and cramping — as does real radiation sickness, which has afflicted no one in Japan, let alone thousands of miles away in the United States.
Why the Outcry?
Fear of radiation is a learned behavior. Moreover, it’s not something we learn from personal experience or observation. We have no way to sense it and must be told by others that we are in danger. As noted above, we receive plenty of information from the media on the dangers of radiation, and this is nothing new. Professor Bernard Cohen of the University of Pittsburgh looked at the New York Times Information Bank, which allows access to numerous publications, and found over the period 1974 to 1978 that there were about 120 stories per year on automobile accidents that killed some 200,000 people. But there were 200 stories per year on radiation that killed no one. Do you know anyone who died or was sickened by radiation? Do you know anybody who knows anybody who was such a victim? The odds are a million to one against it.
Another major reason for the fear is a lack of understanding about what levels of radiation are dangerous and where we might encounter them. Let us establish then how to quantify radiation and relate that to the harm it might cause us. First, though, be aware that we are talking about ionizing radiation from nuclear reactions, X-rays, cosmic rays, or emissions from elements that are naturally radioactive or have been made radioactive from exposure to neutrons in a nuclear reactor. We are not referring to microwave, infrared, or ultraviolet radiation.
Our first hurdle is to understand the units of radiation exposure. Unfortunately, there are two systems and each has different units to express intensity. In the United States the terms more commonly used by medical professionals are the rad and the rem. The rad is a measurement of radiation energy absorbed by matter, while the rem (Roentgen equivalent man) considers not only the amount of radiation, but its biological effect on humans and other animals. For gamma radiation and X-rays, the types of primary interest here, the two terms are equivalent. A rem, however, is a large dose of radiation; hence, to avoid lots of zeros to the right of the decimal point, the term we will be using is the millirem (mrem), one-thousandth of a rem.
The International System (S.I.) — abbreviated from French: Systèm International d´unités — uses two other terms for radiation measurement. The gray (Gy) is equal to 100 rads, and the sievert (Sv) is equal to 100 rems. This is mentioned here because much of the information from other countries and in current news stories is cited in mSv — a millisievert equal to 100 times the exposure of a mrem, i.e., 1 mSv = 100 mrem.†
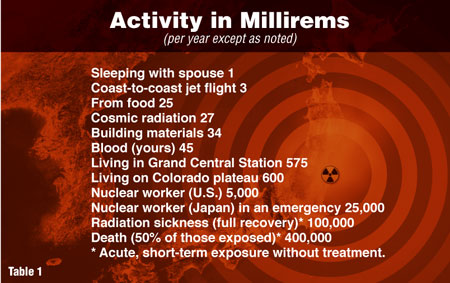
So we now have a unit of measurement — the mrem — that we can use to compare different levels of radiation. Table 1 shows a few examples. Note that one must be exposed to something in the area of 100,000 mrems of radiation in a short period to suffer symptoms of acute radiation exposure, and even more than that to risk death.
Mutation Scares
Survivors of the Hiroshima and Nagasaki bombs were forced participants in a huge epidemiological study of long-term radiation effects. The 86,572 individuals (including pre-born babies) within 10 kilometers (6.2 miles) radius of the blasts were required to carry a “Health Handbook” that recorded even the slightest details of the victim’s health history. Of particular concern to the Japanese was the expectation of thousands of mutations in future births, but such worries were misplaced. While fruit flies that are exposed to radiation are mutated in odd ways (extra legs, eyes, etc.), humans are either not so susceptible, or the degree of mutation is so small as to be lost in the host of normal mutations common to our species. Zbigniew Jaworowski, a member of the UN Scientific Committee on the Effects of Atomic Radiation (UNSCEAR), wrote in Science in July 2001: “In 1958 UNSCEAR had no doubts about major genetic defects in the world population that could be caused by nuclear test fallout, and estimated them as high as 40,000. But now the Committee has learned that even among the children of highly irradiated survivors of atomic bombings no statistically significant genetic damage could be demonstrated (UNSCEAR 2001).”
Delayed Effects
Aside from death and radiation sickness resulting from extremely high doses of radiation, the only other known negative effect of radiation on humans is an increased risk of cancer. While certainly real, this threat seems overblown. Professor John Cameron, of the University of Wisconsin Medical School, points out that there were only about 400 excess cancer deaths in the tens of thousands of exposed individuals in Hiroshima and Nagasaki.
If a large-scale, real-life sampling of radiation survivors didn’t validate hypotheses of skyrocketing cancer rates, though many of the affected people suffered acute radiation exposure, why then do we hear terrifying reports about the number of cancer deaths we can expect from the recent partial meltdown in Japan, where no one has been reported to have faced acute exposure? It is because of a statistical hypothesis known as Linear No Threshold theory, or LNT.
Linear No Threshold theory assumes that there is a linear relationship in the amount of danger posed by increasing levels of radiation. Let’s use aspirin to demonstrate LNT at work. Assume that 100 tablets is a 100-percent fatal dose of aspirin. (That is roughly the case for a 200-pound man.) Linear No Threshold theory would predict that 50 tablets would cause a 50-percent mortality rate, 10 tablets would result in 10-percent mortality, and a single tablet would cause one percent of the users to die. We can pretty well agree that this doesn’t happen with aspirin, but we are told that it does for radiation.
High doses of radiation, for example 100,000 mrem, are carcinogenic and generally follow the LNT. But there is a growing consensus among health professionals that no such risk occurs below 10,000 mrem. Anti-nuclear activists and the media haven’t caught on to this, however, as the following example shows.
Let us assume that the risk of cancer increases by 10 percent for anyone exposed to 100,000 mrem of radiation. If we extrapolate this linearly to zero, then at 10,000 mrem we have a one-percent increase in cancer. At 10 mrem, there would be a 0.001 percent increased risk of cancer. Now comes a hypothetical release of radiation that blankets the country of Japan with a dose of 10 mrem, the U.S. average for 10 days from natural sources. With a population of 127,000,000 people and a mortality of 0.001 percent, the LNT predicts 1,270 increased cancer deaths. Of course the media would pick up on this fact as gospel, needlessly frightening the citizenry with fictitious threats of cancer and death.
Will there be bodies piled in the street? No. Do adherents to LNT have any evidence of these speculated deaths? No. On the order of 19 million cancer deaths would normally occur in the population, so we cannot test their prediction that deaths will increase by 0.001 percent of that total. Since this small number is not statistically significant, who can prove them wrong? (See the related article “The Effects of Low-does Radiation” for the answer.)
Linear No Threshold theory applied to radiation is a shameful lie that causes huge outlays to “protect” the public against trivial amounts of exposure to radiation and is the primary reason behind a fear of nuclear power — promoted, in your correspondent’s opinion, by the radical environmentalists who wield enormous power in our federal and state governments, academia, the media, and, sadly, some of our country’s scientific and professional organizations.
Alpha and Beta Radiation
We have not looked at forms of radiation other than X-rays and gamma rays — both of which are part of the electromagnetic spectrum and essentially the same thing. There are two other forms of ionizing radiation that should be mentioned: alpha and beta particles. The former is a helium nucleus consisting of two protons and two neutrons making it an nuclear giant, while the latter is an electron that depends on a speed near that of light for its energy.
Alpha radiation cannot penetrate more than a few inches of air and is essentially benign unless it is loosed inside the body. Beta radiation cannot penetrate the skin, but can cause “beta burns” that are similar to sunburn. (The only reported radiation “injuries” at the Fukushima complex were caused when three workers waded in contaminated water higher than their boot tops. It is possible they had beta burns on their feet and ankles, but such information has not been made public.)
Neutrons are also always associated with nuclear fission. Though they are not in themselves ionizing particles, they can disrupt cellular activity with a result similar to all the aforementioned forms of radiation.
Isotopes and Half-lives
You may have noticed on news broadcasts that there is usually a number associated with the names of radioactive elements. For example, we hear of Iodine 131 being a dangerous product of fission. How is this different from the iodine our mothers put on our scrapes while we were screaming bloody murder? That iodine was primarily Iodine 127. Both are isotopes of iodine and both hurt when put on a scrape. But 131I (as it is usually designated) is highly radioactive. The Chart of the Elements on the chemistry class wall shows iodine as having the atomic number 53, since all isotopes of iodine have 53 protons. However, each isotope of iodine has a different number of neutrons, and the number of neutrons plus protons, which is known as the atomic mass, is the number assigned to the isotope. 127I has 74 neutrons, while 131I has 78. While chemically identical, the two are radically different in nuclear activity, with the former being stable and the latter radioactive.
Cobalt is another example. Ordinary cobalt, 59Co, is a metal often used to strengthen steel, but after being irradiated with neutrons for 18 months in a reactor, it is converted to highly radioactive 60Co — it is used in industrial radiography.
All radioactive atoms are destined to disintegrate. When an atom of 131I breaks up, it emits a beta particle and gamma rays and is transmuted into stable 131Xe — the noble gas xenon. The new atom is slightly lighter than the iodine atom, with the difference in weight being spent in energy emissions: radioactivity. The time it takes for half of the 131I to be transmuted to xenon is one half-life. For this nuclide the half-life is 8.02 days. After another half-life period, there is a quarter of the original iodine remaining. After 10 half-lives, less than one-tenth of a percent exists. Because of this short half-life, hospitals must be constantly renewing their supply of this useful radionuclide often used in thyroid diagnosis and ablation.
60Co goes through a similar beta decay and is transmuted to stable 60Ni. Its half-life is 5.27 years. Used for such processes as weld inspections and food irradiation, it also must be periodically replaced.
Since 131I gives up its energy much faster than 60Co, you would expect it to be more “radioactive” than the cobalt isotope. This indeed is the case and is related to the activity of the isotope.
Activity
Let us compare the number of disintegrations per second per gram of both the isotopes discussed above. This is called the specific activity of the sample and requires us to understand two additional measuring units. The Curie, named in honor of Madame Curie, who discovered both radium and polonium, is defined as 37 billion disintegrations per second for a single gram. (A gram is less than half the weight of a dime.) It tests the ability of the mind to comprehend the unimaginably small size of atoms when it takes 1,620 years at the beginning rate of 37 billion disintegrations per second for a gram of radium to lose half its mass. (Incidentally, Madame Curie never produced a whole gram of radium during her lifetime of refining and studying the element.)
The unit now used in the S.I. system is the Becquerel, which is defined as one disintegration per second. Hence one Curie (Ci) is equal to 37 billion Becquerels (Bq), not exactly the easiest conversion factor to work with.
For 60Co the activity is about 1,100 Curies! So the atoms of this isotope are changing into stable nickel at the rate of 1,100 times 37 billion, or 41 million billion, disintegrations per second.
But hold on to your hat. 131I checks in at 124,000 Ci, or 4,600 million billion Bq. And 133Te (tellurium) leaves the iodine isotope in the dust, with over a thousand times the disintegrations per second of iodine, and there are other much faster — and more radioactive — isotopes than Tellurium 133 with its half-life of 12.4 minutes.
So how dangerous are substances we usually think of as being highly radioactive, like that evil metal plutonium, which the media portrays as annihilating anyone within shouting distance? 239Pu, with a half-life of 242,100 years, has an activity of 0.063 Ci per gram, or a mere 2,300 billion disintegrations (Bq) per second — one two-millionth the disintegrations of 131I. What about the activity of uranium, which we’re taught to fear so fervently that we insist upon guarding “spent fuel” for hundreds of thousands of years due to its long-term radioactivity? With a half-life of 4.47 billion years, 238U gives up its energy grudgingly at a specific activity of 0.0000003 Ci, or 12,300 Bq. Furthermore, both it and 239Pu are alpha emitters whose radiation is stopped by a sheet of paper. But because uranium is radioactive for a near eternity, it is the poster child for anti-nuclear activists who rely on public ignorance of radiation dangers to undermine the energy industry or who errantly believe that long half-lives mean long-term danger — just the opposite of reality.
It is radioactive substances with short half-lives that are dangerous, but because of their short half-lives, they are not dangerous for long.
Forecast
So what will be the likely outcome of the nuclear meltdown, and the subsequent release of radioactive elements, in Japan? Once pumps are fully operational and structural damage to the nuclear power plant is repaired, the level of radiation from venting the containment vessels and from uncovered spent-fuel cooling ponds will quickly drop, as will airborne particles. Within a few months, the 131I deposited on the ground will decay to zero. Some 137Cs (cesium) will remain detectable on the ground, but at present it appears to be only a tenth the amount of that from the Chernobyl incident — and the amount from Chernobyl was on the same order of magnitude as the natural radionuclides in the soil.
Of course, anti-nuclear activists will predict thousands of cancer deaths based on the LNT, which will not happen, but no matter. Fear is the objective. As we have already seen, the Fukushima “disaster” will become the rallying cry against nuclear power. Few will remember that the plant stayed generally intact despite being hit by an earthquake with more than six times the energy the plant was designed to withstand, plus a tsunami estimated at 49 feet that swept away backup generators 33 feet above sea level. Wonder how those windmills would have stood up.
† The rate of exposure of one microsievert per hour is also commonly used and is equivalent to 876 mrem/year.
Ed Hiserodt is the author of Underexposed: What If Radiation Is Actually Good for You?
Related article: